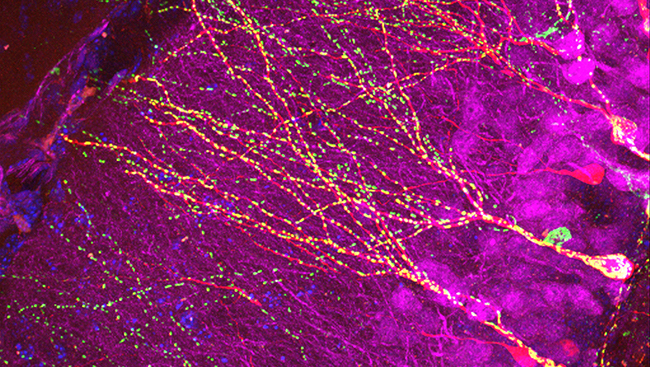
Material below summarizes the article Rapid Mechanically Controlled Rewiring of Neuronal Circuits, published on January 20, 2016, in JNeurosci and authored by Margaret H. Magdesian, G. Monserratt Lopez-Ayon, Megumi Mori, Dominic Boudreau, Alexis Goulet-Hanssens, Ricardo Sanz, Yoichi Miyahara, Christopher J. Barrett, Alyson E. Fournier, Yves De Koninck, and Peter Grütter.
The adult nervous system is composed of billions of neurons that extend axons over substantial distances to reach an appropriate target and build complex networks during development.
Neuronal network function depends on the precise specificity of neuronal connections. Injuries to the nervous system caused by trauma or neurodegenerative diseases usually result in disability and death because it is still not possible to regenerate neurons over long distances and accurately reconnect them with the appropriate target.
In addition, multiple mechanisms limit neuronal regrowth, such as failure to form a new tip capable of guiding the axonal growth and the inhibitory effects of molecules in the scar tissue surrounding the lesion.
Current therapies to promote neuronal regeneration after injury have focused on chemical cues to enhance the intrinsic growth potential of the injured neuron and mask the inhibitors of neuronal extension associated with myelin debris and the glial scar. Despite the development of promising new drugs, achieving long-distance axonal regeneration to establish functional connectivity that mimics the intact nervous system has remained a major challenge.
We overcame these limitations by using tension-stimulated neurite growth to functionally connect two isolated neuronal populations in cultures. With a multidisciplinary team, we developed new micro- and nanotools to rapidly initiate, elongate, and precisely connect new functional neuronal circuits over long distances. Previous work in our lab has shown that poly-D-lysine coated beads (PDL-beads) induce the formation of presynaptic boutons. We also showed that when the PDL-bead is mechanically pulled away after presynaptic differentiation, the synaptic protein cluster follows the bead initiating a new neurite.
In this study, we investigated the limits of extension and functionality of the new neurite. We developed silicone microfluidic devices to grow rat hippocampal neurons for 2-3 weeks in two isolated populations on a glass coverslip. The microfluidic devices were removed and PDL-beads were placed on neurites. After adhesion of PDL-beads to neurites, we used atomic force microscopy or micromanipulation techniques to pull the PDL-bead-neurite complex. As the PDL-bead-neurite complex moved, a new neurite was formed. As we pulled, we elongated the new neurite for several hundreds of microns (up to 840 µm, a limit set by our instrumentation) and guided it towards the second neuronal population in less than one hour. We thus initiated and extended a new neurite from one neuronal population and precisely connected it to a second neuronal population several hundreds of microns apart in less than two hours.
To test the functionality and the type of connection, we put together an electrophysiology setup enabling paired recordings. This allowed us to first manipulate a connection between the two isolated populations and then investigate signal transmission through the newly formed connection by stimulating any neuron on one population and performing whole cell patch clamp recordings on a neuron in the second population.
As we hoped, spikes in the first population were followed by an increase in postsynaptic currents in the second population, suggesting that the two populations were now connected. These results indicate that the newly formed neurites can establish functional synaptic connections between the two previously isolated populations.
This work shows that by using standard micromanipulation techniques, it is possible to create new neurites, elongate them for hundreds of microns in less than one hour, and functionally connect the new neurites to a desired target to re-wire neuronal networks. Our results also unexpectedly show that the intrinsic capacity of these neurites for elongation is much faster than previously thought. The extension rates achieved are faster than 20 microns per minute over millimeter-scale distances, and functional connections are established.
Our proposed mechanical approach bypasses slow chemical strategies and thus represents a paradigm shift for therapeutic developments to restore neuronal connectivity after injury. Our results have direct implication for therapies that aim to reconnect neuronal circuits after trauma or in neurodegenerative diseases. The tools presented here can be used to create systematically engineered neuronal networks as novel bioassay platforms for drug discovery and target validation. This technique also enables micro-neuroengineering of artificial neural networks in culture to test hypotheses about circuit function and dynamics, opening the door for the direct wiring of robust brain-machine interfaces.
We believe that our findings will have a major impact on regenerative medicine and neuro-engineering approaches.
Visit JNeurosci to read the original article and explore other content. Read other summaries of JNeurosci and eNeuro papers in the Neuronline collection SfN Journals: Research Article Summaries.
Rapid Mechanically Controlled Rewiring of Neuronal Circuits. Margaret H. Magdesian, G. Monserratt Lopez-Ayon, Megumi Mori, Dominic Boudreau, Alexis Goulet-Hanssens, Ricardo Sanz, Yoichi Miyahara, Christopher J. Barrett, Alyson E. Fournier, Yves De Koninck, Peter Grütter. The Journal of Neuroscience Jan 2016, 36(3): 979 –987; DOI: 10.1523/JNEUROSCI.1667-15.2016