A Specific Population of Immature Interneurons Shapes the Dynamics of Network Activity Throughout the Developing Hippocampus
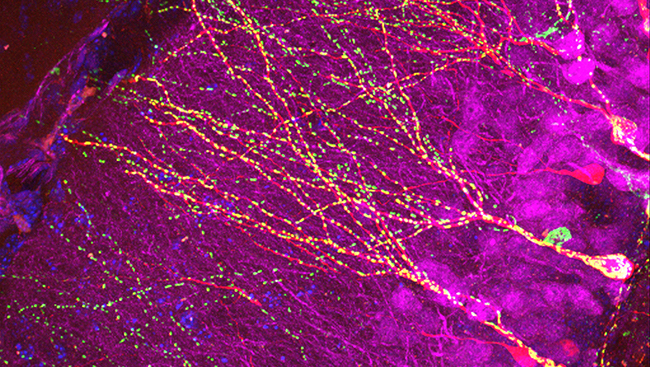
Material below summarizes the article Interneurons Differentially Contribute to Spontaneous Network Activity in the Developing Hippocampus Dependent on Their Embryonic Lineage, published on March 2, 2016, in JNeurosci and authored by Jason C. Wester and Chris J. McBain.
A key feature of neural circuits is their ability to internally generate spontaneous bursts of network activity that can propagate as a wave over long distances. In mature brains, such activity occurs predominantly during slow-wave sleep, and there is mounting evidence that it serves to reactivate and strengthen synaptic connections formed earlier during learning and exploration.
Amazingly, very similar patterns of activity occur throughout the immature brain during the development of the first functional synapses. Thus, even in the absence of mature external sensory input, the nervous system produces internally generated activity in order to guide the formation of circuits that can be later sculpted by experience.
In the neocortex and hippocampus, the first synaptic connections to form are from interneurons that use the neurotransmitter GABA. While in the mature nervous system GABAergic transmission is inhibitory, during early development it is excitatory. Thus, immature interneurons importantly contribute to generating local network activity and play a crucial role in promoting the establishment of local synapses and circuits.
Interneurons are an incredibly diverse group of cells that target different regions of excitatory pyramidal cells in order to shape their output. Furthermore, different subtypes can be distinguished based on their differential expression of specific protein and peptides.
In spite of their diversity, cortical interneurons can be segregated into two distinct subsets based on their embryonic origin from either the medial or caudal ganglionic eminences (MGE and CGE). MGE-derived interneurons include parvalbumin-containing, somatostatin-containing, and NPY/nNOS-positive containing subtypes. CGE-derived interneurons include cholecystokinin-containing, VIP-containing, and NPY/nNOS-negative cell types.
We decided to test the hypothesis that these two groups of interneurons play different roles in promoting early spontaneous network activity in the developing hippocampus, referred to as giant depolarizing potentials (GDPs).
We used whole-cell patch clamp recordings of pyramidal cells and interneurons to monitor GDPs in slices of mouse neonatal hippocampus prepared 5-7 days after birth. We focused our recordings in the CA1 region where excitatory pyramidal cells are only sparsely interconnected and thus interneurons provide the majority of the local circuit excitatory drive.
Our strategy was to use optogenetics to transiently remove either MGE- or CGE-derived interneurons from the local circuit and assess the impact on spontaneously occurring GDPs. Specifically, we took advantage of transgenic mouse lines to express archearhodopsin (Arch) selectively in either MGE- or CGE-derived interneurons in order to inhibit these cells with light.
We recorded spontaneous GDPs during three contiguous 10-second time bins before, during, and after a light stimulus to activate Arch. We then quantified the number of GDPs that occurred during each of those bins recorded over several trials.
We found that Arch-mediated inhibition of MGE-derived interneurons dramatically reduced the number of GDPs relative to the periods immediately before and after the light stimulus. In fact, in some slices, GDPs were completely abolished during the stimulus. In stark contrast, inhibition of CGE-derived interneurons resulted in only a moderate reduction in the number GDPs, and in no cases were they completely abolished.
Furthermore, when MGE-derived interneurons were inhibited, GDPs were reliably generated approximately 200 milliseconds after the end of the light stimulus. We referred to these as “rebound GDPs,” which were likely due to expression of the h-current, a depolarizing current that it is activated by hyperpolarization. Thus, when the light stimulus was removed, a population of MGE-derived interneurons was depolarized and in turn triggered a GDP. Importantly, rebound GDPs were only rarely observed when CGE-derived interneurons were inhibited. Thus, our data unambiguously show that MGE-derived interneurons play a dominant role in GDP generation in hippocampal CA1.
We next investigated the circuit mechanisms underlying the different contributions of MGE- versus CGE-derived interneurons by performing dual whole-cell patch recordings to test for synaptic connections between these cells and neighboring pyramidal cells (PCs).
We found that immature MGE-derived interneurons were densely interconnected with neighboring PCs, sending and receiving synaptic connections with high probability and in some cases forming reciprocal connections. In contrast, connections between CGE-derived interneurons and PCs were very rarely observed. Finally, we found that the axons of these immature MGE-derived interneurons commonly targeted the perisomatic region of PCs; thus, they provide input near the site of action potential initiation. In contrast, CGE-derived interneurons rarely innervated this region, and instead commonly targeted the more distal dendrites of PCs.
Although we performed the above experiments in region CA1, GDPs are generated throughout the hippocampal formation. In particular, neighboring CA3 is likely the primary generator of GDPs: In this region, there is dense connectivity among pyramidal cells in addition to excitation from interneurons. Furthermore, the dominant excitatory projection in mature hippocampus is from CA3 to CA1; thus, it has been generally assumed that GDPs are initiated in CA3 and then transmitted to CA1 as a wave.
We tested the influence of MGE-derived interneurons throughout the hippocampus by recording in CA1 and CA3 simultaneously and selectively stimulating each region with light to activate Arch.
As expected, inhibiting MGE interneurons in CA3 resulted in a reduction in spontaneous GDP frequency in both CA3 and CA1, consistent with the idea that activity in CA1 mainly follows that in CA3. However, surprisingly, we found that inhibiting MGE interneurons in CA1 also reduced GDPs in CA3. This strongly suggests that there is crosstalk between these regions to coordinate spontaneous activity across the developing hippocampus. Supporting this conclusion, we observed that rebound GDPs were consistently triggered at the site of the light stimulus. Thus, light in CA1 triggered GDPs that then propagated to CA3, while light in CA3 triggered GDPs that then propagated to CA1.
Our data demonstrate for the first time that the subset of interneurons specifically derived from the MGE act as a key regulator of spontaneous network activity throughout the developing hippocampus. Furthermore, they are likely the primary driver of GDPs in CA1. Thus, these interneurons are crucial for promoting the development of circuits involved in spatial working memory and navigation in mature animals.
Visit JNeurosci to read the original article and explore other content. Read other summaries of JNeurosci and eNeuro papers in the Neuronline collection SfN Journals: Research Article Summaries.
Interneurons Differentially Contribute to Spontaneous Network Activity in the Developing Hippocampus Dependent on Their Embryonic Lineage. Jason C. Wester and Chris J. McBain. The Journal of Neuroscience March 2016, 36(9): 2646-2662; DOI: 10.1523/JNEUROSCI.4000-15.2016