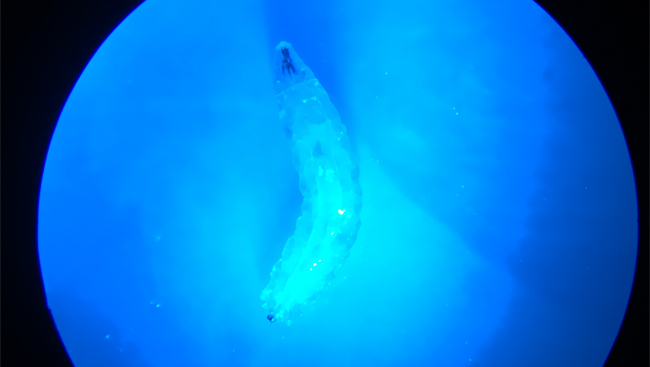
An Accessible Approach to Optogenetics in the Classroom Using Drosophila
By Heather J. Rhodes
Optogenetics is such an important and exciting tool in neuroscience research that I decided to add it to my undergraduate courses at Denison University, the liberal arts college where I teach.
Although I’d never used optogenetics as part of my own research, I’ve now successfully added a Drosophila optogenetics lab to one of my courses with low costs, reasonable preparation, and meaningful impact.
Students benefited from a hands on experience with in vivo optogenetics, and they even designed their own experiments.
In this article, and Implementing Optogenetics in the Classroom Part Two: C. elegans, I share my advice for adopting optogenetics approaches for teaching purposes.
Why Drosophila
Drosophila are an effective model system for optogenetics. You can order a variety of genetic lines and they are relatively easy to raise and cross, allowing you to express channel rhodopsin or other effectors (halorhodopsin or TRPA1, for instance) in specific subsets of neurons.
Because the Drosophila larva are translucent, you can activate channel rhodopsin in vivo with an LED light source while observing behavior. In the lab I describe below, larvae exhibit an instantaneous rigid paralysis when blue light is applied. This is dramatic and easy to measure.
Furthermore, many schools already use Drosophila for genetics or other courses, meaning you may already have most of the supplies you need right down the hall.
Exploring the Literature
My first foray into optogenetics was a two part lab in an upper-level neurophysiology class. I had seen papers describing undergraduate labs (Pulver et al., 2011, Berni et al., 2010, and Titlow et al., 2015), but these involved a level of complexity I wasn’t ready for as a beginner and tools I didn’t have in my teaching lab (such as fluorescent microscopy).
When I found a paper targeted at a high school audience (Titlow et al., 2014), I felt I’d found something I could use. Titlow and colleagues provided supply lists and instructions for constructing a simple and inexpensive blue light source (a blue LED powered by a 9V battery, taped to a standard microscope eyepiece to focus the light).
Setting Up the Experiments
Using this guidance, I knew I could execute the experiment with nothing more than my existing dissecting scopes. A colleague who works with Drosophila showed me how to rear my flies, then let me use his space and equipment to do so.
If you don’t have local help, there are many online resources to get started with Drosophila, including here and here.
Based on Titlow et al., I wrote a two part lab exercise (download the Drosophila lab handout).
In the first lab session, students learned about optogenetics and the particular construct we utilized: a fly line that expressed channel rhodopsin in all glutamatergic neurons, including all motor neurons.
Students developed a hypothesis about what would happen when they activated all glutamatergic neurons simultaneously. They developed a simple method to quantify larval movement and then activated the channel rhodopsin and observed the results.
This was successful, with obvious results — tetanic paralysis — that the students could easily observe, repeat, share, and discuss.
As soon as the light was removed, locomotion resumed. Fly larvae must be fed all-trans retinol (ATR) in their food to produce functional channel rhodopsin, so a control group of genetically identical larva without ATR fail to show the paralysis in response to blue light.
Challenging Students to Design an Experiment
After completing this inquiry-based exploration, students were then challenged to design their own experiment for the second part of the lab.
They could choose four possible fly crosses, using either a cholinergic or glutamatergic promoter with either channel rhodopsin or a heat sensitive, excitatory ion channel (TRPA1) as the effector gene (see Berni et al., 2010).
Working in groups of 3-4, students proposed an experimental question, developed a hypothesis with a clear rationale, designed a treatment, and specified how they would quantify and collect data.
I prepared the requisite crosses, and students were able to perform their experiments two weeks later.
Students designed interesting experiments and asked questions such as: Will activation of cholinergic neurons with channel rhodopsin disrupt olfactory responses, and will activation of cholinergic neurons with TRPA1 influence photophobic behavior?
Other students tested how different wavelengths of light activated channel rhodopsin or explored how different temperatures induced TRPA1 activity. By letting students design their own experiments, they were given additional experience with the technique, ownership of the work they did, and a sense of the wide range of applications for this technology.
What Students Gained
I found no substantial downsides to this lab. Setting up fly crosses and rearing flies were the only significant efforts, and a strong lab teaching assistant could manage that workload.
The upsides, on the other hand, were tremendous. Students developed an understanding of what optogenetics entails and how it can be used in neuroscience. Given the range of flies available, this lab could also be structured to explore how particular groups of neurons or other neurotransmitter systems contribute to behavior.
I look forward to running this lab again, and encourage others to use it with your own students. All you really need is a microscope, some blue LEDs, and the right flies.
Download the Drosophila lab handout.
Visit the Community forum for all eight modules to share your insights and best practices, ask questions, and engage with other training series’ participants.
Complete this short survey to provide valuable feedback about Module 5 to SfN and the series faculty.