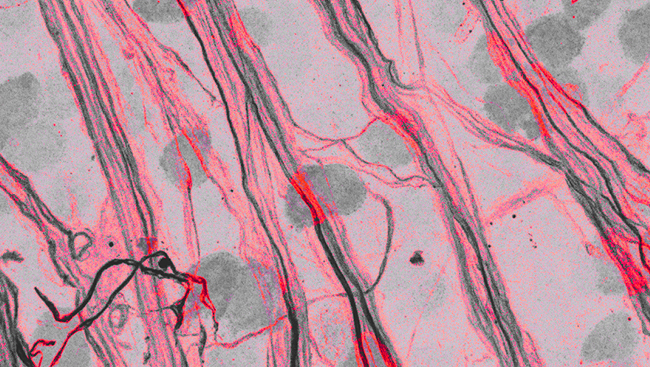
Material below is adapted from the SfN Short Course Novel Technologies for Single-Cell Resolution Whole-Transcriptome Analysis in CNS Tissue, by Evan Macosko, MD, PhD. Short Courses are day-long scientific trainings on emerging neuroscience topics and research techniques held the day before SfN’s annual meeting.
Scientists do not always agree about how many different types of cells are found in the mammalian brain, nor do they understand all the variation in gene expression that exists even within well-studied categories of cells. But innovations that allow for an efficient look at the whole transcriptomes of tens of thousands of single cells at a time bring the answers to these questions closer than they have ever been before.
Isolating individual cells presents technical challenges. Historically, scientists have picked out single cells by hand, which makes it easy to visually confirm that only one cell is selected, but is time and labor intensive. Sorting cells with flow cytometry or microfluidic techniques speeds up the process. For instance, one method allows for the separation of thousands of cells per hour. But cells that stick together, called doublets, can confound these faster methods. To avoid doublets, researchers can dilute cell suspensions, visually spot check dispersed cells, and introduce cells of another species, which enables quantification of doublet rates after RNA sequencing.
Another hurdle to single cell transcriptome analysis is the relatively small amount of RNA found in an individual cell, but techniques exist to amplify tiny quantities of starting material. One complementary DNA (cDNA) library preparation method involves an oligo dT primer bound to an RNA polymerase derived from the T7 bacteriophage. The T7 polymerase synthesizes cDNA from all polyadenylated messenger RNA (mRNA), and the cDNA is then amplified via reverse transcription. Another common strategy involves the use of reverse transcriptase from Moloney murine leukemia virus to generate and amplify cDNA.
Isolating individual cells, preparing libraries, and running the RNA sequencing reactions can be time intensive, especially if researchers must complete all these steps for each cell. One way to overcome this obstacle is to introduce molecular barcodes that are specific to single cells. These molecular barcodes are part of the oligo dT primer, so they bind to mRNA. Then cells are lysed and cDNA library generation, amplification, and sequencing for multiple cells can take place in a single experiment. In one strategy, molecular barcodes are specific to an individual cell, but each primer also contains a unique molecular identifier, which allows for quantification of individual mRNAs.
Whenever scientists attempt RNA sequencing of a group of individual cells, they must consider how efficiently they have captured the RNA in a single cell. Only a portion of total RNA in a cell is polyadenylated mRNA, and most methods capture about a tenth of that mRNA. Low abundance transcripts are likely missed in single cells, but may be detected if many individual cells of the same type are sequenced.
Another question that arises in RNA sequencing is how variable the results are across repeated experiments. It is often necessary to sequence several groups of cells on different days in order to generate experimental power, and it is possible to compare results from several sessions to insure consistency between them.
Analyzing the whole transcriptomes of single cells used to be painstaking and inefficient, but the technical advances discussed here have made new types of single cell transcriptome analysis possible. Scientists have analyzed groups of individual cells from the mammalian retina, spinal cord, and cortex for transcriptional variation. They may be able to use data gained from these experiments to better connect cell type, gene expression, and disease risk in the future. Another exciting application of single cell RNA sequencing is to examine the effects of drugs, pathogens, and genetic mutations on individual cells and cell types.
Researchers are also at work on improvements to single cell RNA sequencing, including strategies to obtain gene expression profiles from individual cells in fixed and frozen tissue. The development of methods that do not rely on the destruction of tissue would also allow scientists to better understand the relationships between gene expression in nearby cells. Finally, combining epigenetic and genomic analyses with single cell RNA sequencing could yield biological insights about how these levels of regulation are connected within cells and also how they create differences across types of cells.