Precise Spike Patterns in Cortical Neurons Encode for Touch Stimuli
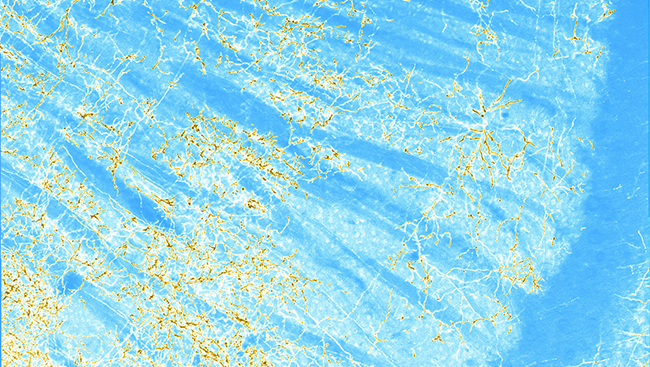
Material below summarizes the article Refractoriness Accounts for Variable Spike Burst Responses in Somatosensory Cortex, published on August 14, 2017, in eNeuro and authored by Bartosz Teleńczuk, Richard Kempter, Gabriel Curio, and Alain Destexhe.
In the cortical area responsible for the processing of touch, the primary somatosensory cortex (S1) neurons can respond to touch stimuli with a very high precision. This is measured by repeating the same stimulus many times and recording neurons in S1 using a micro-electrode.
This was shown in macaque monkeys. Electrically stimulating the median nerve (which relays the touch information from the hand) at the wrist leads to the firing of high-frequency bursts of action potentials in the primary somatosensory cortex. Remarkably, this pattern of firing is repeatable with sub-millisecond precision.
Due to this precision, the responses averaged over multiple trials form a multi-peaked, post-stimulus time histogram; each of its peaks corresponds to a single spike within the burst. Therefore, such bursts are also population phenomena that are visible macroscopically in the high-frequency epidural EEG (hf-EEG) recordings.
We reproduced this result with simple models. We found feedforward models of spiking neurons can account for this phenomenon, even in the presence of noise.
The model consists of noisy (Poisson) neurons that receive strong time-dependent inputs and become refractory for a short period of time after emitting a spike. When the burst is triggered by a strong excitatory input, the model reproduces the precise spike pattern, but only if refractoriness is taken into account. Fitting this model to the experimental data shows it can account for a large number of the response statistics (post-stimulus time histogram, interspike interval distribution, correlations between consecutive interspike intervals).
The model can also reproduce the appearance of so-called temporal spike patterns consisting of a series of spikes and intervening silences.
For example, two spike patterns can be obtained: a series of three consecutive spikes aligned to the peaks of the post-stimulus time histogram (a triplet) or a pattern in which the middle spike is missing (a doublet). While the amplitude of the synaptic activity received by the neuron is modulated, the neuron can show a differential preference for eliciting any of these patterns.
Interestingly, these single-neuron spike patterns are also associated with specific population markers (post-stimulus time histogram), giving rise to a significant correlation between micro- and macro-scopic neuronal responses. We observed similar correlations in the simultaneous single-neuron and epidural EEG recordings in macaques.
In a separate model based on the integrative property of the neuronal membrane coupled with cortical and thalamocortical connectivity (leaky integrate-and-fire neuron in the so-called high-conductance state) noisy response of the neuron result from the continuous synaptic bombardment generated by the ongoing activity in the cortex. In this model, the refractoriness can be associated with the time necessary to reach the steady membrane potential after hyperpolarization following the spike emission.
In summary, our study shows touch information is coded as very precise spike patterns and the exact pattern is modulated by the network activity. As the refractoriness and time-dependent inputs are ubiquitous features of cortical neurons, such temporal spike patterns may also be present in other brain areas. Therefore, the coding principles we demonstrated could be used by other brain areas for the processing of sensory information with high temporal resolution.
Visit eNeuro to read the original article and explore other content. Read other summaries of JNeurosci and eNeuro papers in the Neuronline collection SfN Journals: Research Article Summaries.
Refractoriness Accounts for Variable Spike Burst Responses in Somatosensory Cortex. Bartosz Teleńczuk, Richard Kempter, Gabriel Curio, and Alain Destexhe. eNeuro Aug 2017, 4 (4) ENEURO.0173-17.2017; DOI:https://doi.org/10.1523/ENEURO.0173-17.2017