Measurement of Retinal Blood Flow Using Fluorescently Labeled Red Blood Cells
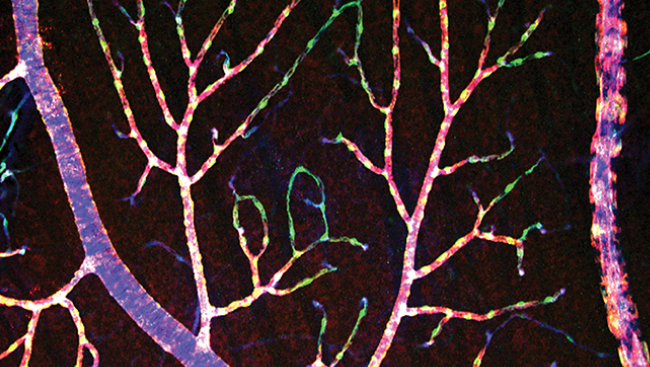
Material below summarizes the article Measurement of Retinal Blood Flow Using Fluorescently Labeled Red Blood Cells, published on April 29, 2015, in eNeuro and authored by Tess E. Kornfield and Eric A. Newman.
Our brain is a hungry organ. Although it accounts for only two percent of our body weight, the brain consumes 20 percent of the oxygen and 25 percent of the total nutrients that our body utilizes. The brain has an extensive blood supply to feed itself. Interruption of this supply, even for just a few minutes, can damage the brain permanently. The brain’s vasculature has many mechanisms to ensure that the blood supply is always sufficient. If the body’s blood pressure drops, the brain’s blood vessels dilate to make up the difference. If neurons in a brain region are particularly active, the vessels in that region dilate to bring extra blood to feed those neurons.
In order to understand the mechanisms that ensure that the brain is adequately supplied with oxygen and nutrients, it is necessary to accurately measure blood flow in the brain.
Many techniques have been developed to measure changes in blood flow, that is, how much blood flow increases or decreases relative to baseline. Some of these techniques are based on the Doppler effect, which measures the velocity of blood by shining a laser beam on vessels and detecting a change in the wavelength of the light after it is reflected off of moving blood cells. However, Doppler measurements cannot easily measure absolute blood flow, which is expressed in units of milliliters per second.
We have developed a simple technique for measuring absolute blood flow by counting the number of red blood cells (RBCs) that pass through a vessel. The technique has several advantages over other methods of measuring absolute blood flow, including: The technique can accurately measure absolute blood flow in a wide range of vessel sizes, from 5 mm capillaries up to 70 mm venules, and the technique measures blood flow directly and does not rely on complex assumptions or calculations.
Our technique tracks the passage of fluorescently labeled red blood cells (fRBCs) using a high-speed confocal microscope. To make the fRBCs, we withdraw a small volume of blood from an animal, incubate the RBCs with a fluorescent dye, and re-inject the labeled blood back into the animal. The fRBCs make up 0.1-1.8 percent of all the RBCs in the blood. We then scan a blood vessel with the microscope’s laser beam that is directed back and forth across the width of the vessel. When a fRBC passes the laser beam, it is detected as a spot of light. Because RBCs travel quickly through large vessels (~20 mm/s) the laser beam must scan back and forth very quickly so that all the fRBCs are detected. We use a scan rate of 4,200 scans/s. A computer program automatically counts the fRBCs and we end up with a measure of fRBC flux, or the number of cells passing through the vessel per second.
Once we have counted the fRBCs passing through a vessel, we easily convert it to a measure of blood flow. All we need to know is the number of fRBCs per volume of blood. We do this by withdrawing a small amount of blood from the animal and counting the number of fRBCs in a known blood volume. This is done with a hemocytometer, which is a microscope slide that is engineered to hold a precise volume of blood. Blood flow is then calculated with the equation: Blood flow (ml/s) = fRBC flux (cells/s) / number of fRBCs per volume (cells/ml).
We have tested our method of measuring absolute blood flow in the retina, which is part of the central nervous system. Blood vessels in the retina are viewed with a confocal microscope through the cornea and lens of an anesthetized rat. We determined the accuracy of our method in a number of ways.
First, we compared blood flow calculated by counting fRBCs to an independent measurement of blood flow obtained by measuring both RBC velocity and the diameter of the vessel (Blood flow equals blood velocity times the cross-sectional area of the vessel). We found that the two methods of measuring absolute blood flow yielded nearly identical results for measurements in vessels varying in diameter from 5 to 70 mm.
We also tested our method by measuring absolute blood flow at a vessel branch point, where a parent vessel splits into two daughter vessels. We found that flow in the parent vessel equaled the sum of the flows in the daughter vessels, as expected.
Our method can also be used to measure the total blood flow in the retina. All of the blood vessels in the retina originate from a single vessel called the central retinal artery. To measure total retinal blood flow, we simply added up the blood flow in each of the arterioles branching from the central retinal artery. This equaled the sum of the flows in each of the venules exiting the retina though the central retinal vein. This is as it should be because all of the blood entering the retina through the arterioles must exit the retina through the venules.
Finally, we found that our method of measuring absolute blood flow was sensitive to changes in flow. When an experimental animal breathed oxygen-enriched air (causing hyperoxia), blood flow decreased. In contrast, when an animal breathed carbon dioxide-enriched air (causing hypercapnia), blood flow increased. Our technique was sensitive enough to detect these expected physiological responses.
Our results demonstrate that absolute blood flow can be measured simply and accurately in vessels having a wide range of sizes using a straightforward method that relies on few assumptions. The method can be used to measure blood flow in the brain and in other body organs as well as the retina. We believe that the method will prove useful in future studies of blood flow and metabolism in the retina and the brain.
Visit eNeuro to read the original article and explore other content. Read other summaries of JNeurosci and eNeuro papers in the Neuronline collection SfN Journals: Research Article Summaries.
Measurement of Retinal Blood Flow Using Fluorescently Labeled Red Blood Cells. Tess E. Kornfield and Eric A. Newman. eNeuro Mar 2015, 2 (2). DOI: 10.1523/ENEURO.0058-14.2015