Distinct Rules Govern Co-Modulation of Circuit Components by Two Modulators
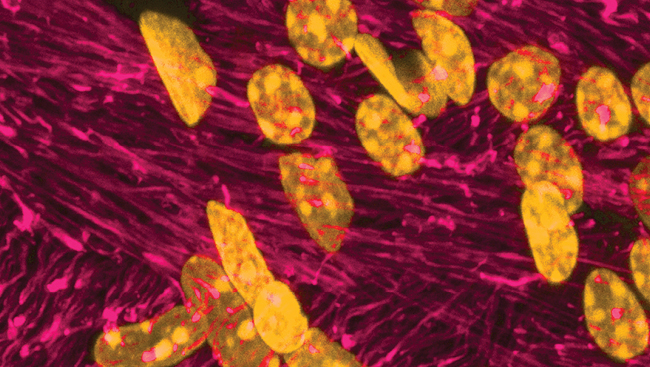
Material below summarizes the article Distinct Co-Modulation Rules of Synapses and Voltage-Gated Currents Coordinate Interactions of Multiple Neuromodulators, published on October 3, 2018, in JNeurosci and authored by Xinping Li, Dirk Bucher, and Farzan Nadim.
All nervous systems adapt to changes in the environment and the internal state of the animal. Such flexibility is essential to producing behaviors in different contexts.
Much of this plasticity arises through the actions of neuromodulators, which actively reshape the activity and output of neuronal circuits by modifying neuronal excitability and synaptic transmission, typically by activating distinct G protein-coupled receptor-mediated pathways.
Prominent examples include modulatory actions mediating distinct brain or behavioral states, such as the function of serotonin in regulating mood, or the sleep-wake cycles mediated by monoamines and peptides, such as orexin.
Although it is tempting to equate the actions of individual neuromodulators with specific behaviors or even brain states, neural circuits are not exposed to neuromodulators one at a time.
Rather, any part of the nervous system is continuously subjected to the actions of multiple neuromodulators released from distinct modulatory neurons, or often co-released from the same terminals. For example, hypothalamic neurons that promote wakefulness co-release orexin and dynorphins, as well as glutamate.
Nevertheless, the implicit assumption that a single dominant neuromodulator is responsible for a specific behavior or brain state is evident in common experimental paradigms to assess the effect of manipulating an individual neuromodulator on a neuron or circuit. Consequently, how different combinations of modulators act in concert to sculpt circuit activity is poorly understood.
Different neuromodulators may act on different subcellular targets in the same neuron, or converge on the same synaptic component or ion channel. Co-modulators can act synergistically or oppose one another, and these actions may be through converging or parallel signaling pathways.
To understand how multiple modulators modify circuits, it is important to reveal the rules of co-modulation at the level of individual circuit components, principles governing the interactions of modulators targeting the same ion channel or synapse.
As a first step towards this goal, we focused on the quantitative rules of co-modulation of synaptic and voltage-gated currents by convergent modulators. The simplest assumption is the combined action of such modulators is the linear sum of their individual effects, up to the saturation level.
We examined the validity of this simple rule in the pyloric circuit of the crab stomatogastric nervous system. We chose this model system because a long history of studies has provided extensive knowledge of the influence of neuromodulators on this central pattern generating circuit.
The pyloric circuit consists of a small number of experimentally accessible identified neurons, and the connectivity diagram is well known. The circuit produces a stereotyped stable rhythmic bursting pattern, and it is modulated by an astounding number of neurotransmitters and hormones. The effects of several individual modulators on the circuit and its component neurons and synapses have been characterized.
Notably, monoamines, such as dopamine and serotonin, target multiple ionic currents in pyloric neurons and can have opposing effects on the same current type in different neurons. In contrast, excitatory peptide modulators seem to act in a simpler manner by converging to activate a single voltage-gated ionic current: the modulator-activated inward current, IMI.
Not all neurons in the circuit, however, have receptors for all these peptides. This circuit-level divergence means different combinations of neurons are modulated by each peptide, resulting in different modulator-specific versions of circuit output activity. Divergence also occurs through distinct dose-dependent IMI activation by different peptides.
Modulators also influence the strength and dynamics of pyloric synapses. As with voltage-gated ionic currents, monoamines often have cell-specific and opposing effects on the strengths of pyloric synapses.
The effects of peptides on pyloric synapses has not been explored as thoroughly. In the few studies where these effects have been characterized, excitatory peptides have been shown to strengthen target synapses, but it is unknown whether these effects are achieved through the same signaling pathway that influences IMI activation.
Despite the vast number of known modulators of the pyloric circuit and the extensive exploration of the circuit- and component-level effects of numerous individual modulators, only a handful of studies have explicitly characterized co-modulation.
To test co-modulation, we focused on two individually identifiable neurons, the pyloric dilator (PD) and the lateral pyloric (LP), and their reciprocal synapses, and two peptides with known distinct actions at the circuit level.
We found both peptides enhanced the strength of either synapse. As had been described before, in the LP neuron, both peptides activated IMI. We voltage-clamped the neurons simultaneously and in each experiment characterized the dose-dependent effect of either peptide on the LP-to-PD and PD-to-LP synapses, and on the IMI level in the LP neuron. We then determined the degree of occlusion at saturating concentrations. We used these dose-response curves to predict co-modulation effects at any combination of concentrations, under the assumption of linear summation up to saturation.
To test the predictions, we then measured the combined effects of the two peptides at 20 different combinations of concentrations. We found both synapses obeyed the simple additive co-modulation rule almost perfectly, indicating simple convergence of the signaling pathway to subcellular targets.
Yet, in the same experiments, this rule could not describe the co-modulatory activation of IMI in the LP neuron. At every concentration combination examined, the combined effect of the two modulators was smaller than the linear sum of the individual effects. At low concentrations, the difference was substantial, and only near saturation levels did the simple additive rule produce a reasonable prediction of the co-modulatory effect.
We were able to explain that IMI co-modulation obeys a sublinear rule when we assumed that the secondary effectors downstream of the two receptor types inhibit one another. Indeed, simply including mutual inhibition of the IMI activation pathways from each modulator receptor produced an almost perfect prediction of the co-modulatory effects.
Perhaps the most general implications for our understanding of co-modulation arise from the possibility the combined effects of modulators can diverge even within a single neuron.
Our findings suggest the intracellular second-messenger-mediated pathway leading to the activation of IMI is distinct from the pathway leading to synapse enhancement, as the effects on synaptic currents combined linearly, but the effects on IMI combined sublinearly.
Considering the complexity of intracellular signaling cascades and their possible compartmentalization into microdomains, it is not difficult to imagine a divergence of the two pathways downstream of the receptors.
Such divergence of co-modulatory rules could provide an additional level of flexibility, as the effects of a modulator on distinct targets can be modified by even low concentrations of a co-modulator. In our case, each peptide modulator would enhance the synapses and IMI, but in the presence of both peptides, the synaptic enhancement would be greatly accentuated.
It remains to be shown to what degree co-modulation rules remain consistent across different circuit neurons and synapses and across different neuromodulators, and how such rules at the cellular level translate into concentration-dependent interactions of co-modulators on circuit activity.
However, these results underline the importance of a detailed quantitative assessment of the rules that govern co-modulation across realistic combinations of modulators at different concentrations.
As we go beyond what can be gleaned from anatomical circuit connectivity, quantitative models of circuit dynamics and function become more ubiquitous in the endeavor to understand the neural basis of behavior.
To accurately represent the plasticity of biological neural circuits, such models will have to include the rules governing the combined actions of neuromodulators that reshape circuit activity in different behavioral contexts.
Visit JNeurosci to read the original article and explore other content. Read other summaries of JNeurosci and eNeuro papers in the Neuronline collection SfN Journals: Research Article Summaries.
Distinct Co-Modulation Rules of Synapses and Voltage-Gated Currents Coordinate Interactions of Multiple Neuromodulators. Xinping Li, Dirk Bucher, Farzan Nadim. JNeurosci Oct 2018, 38 (40) 8549–8562; DOI: 10.1523/JNEUROSCI.1117-18.2018